Special Report: The challenges of offshore grids
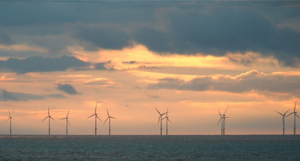
Wind turbines
The high potential of offshore wind resources, a need for increased electrical interconnection at European level, and reduction of the CO2 emissions from oil and gas platforms are the main drivers of the Super Grid development in the North Sea. This will be a pioneering project employing innovative technologies and solutions, such as large, meshed HVDC systems. The Norwegian University of Science and Technology (NTNU) is at the forefront of research and experimental validation of concepts, components and systems that will make the North Sea Super Grid a reality in the coming decades.
The increasing energy consumption at the global level, the progressive depletion of fossil fuels, and a deep concern for environmental sustainability are urging the widespread adoption of renewable energy solutions. The exploitation of photovoltaic and wind energy onshore is already consolidated in several countries, and increasing attention is now being focused on the oceans, which are still a largely untapped energy resource. The potential of so-called ‘blue energy’ is huge: wind resources offshore are steadier and more powerful than onshore winds, and their exploitation would be possible with larger turbines, without severe limitations due to road transportation, visual impact and acoustic noise restrictions. Other types of offshore energy generators such as wave and tidal energy converters, although still at an earlier stage of development, could increase power production from ocean resources in the coming years. This will contribute to the diversification of the energy portfolio, increasing energy security at European and international level.
Offshore renewables in the North Sea could provide further benefits through their integration with offshore oil and gas installations. Currently, gas or diesel generators operating at low efficiency and with high CO2 emissions locally produce the electricity required by oil and gas platforms. The possibility of integrating such installations with renewable offshore energy generators, or, alternatively, to supply them from shore, would cut their corresponding CO2 emissions by one order of magnitude.
Electric grid offshore
The exploitation of offshore energy sources – both to supply electricity to offshore oil and gas platforms and to cover the energy needs of onshore load centres – relies on the capability to efficiently transmit electrical power from the point of generation to the point of final consumption.
However, the creation of offshore electric infrastructures poses several additional challenges compared to traditional onshore power systems. The deployment of offshore wind farms of increasing size in the last two decades has already provided an example of the materials and technologies that are suitable for such a harsh environment. It has also indicated which operational, maintenance and reliability requirements impact significantly on the final cost of offshore energy.
On the other hand, the possibility of systematically integrating offshore energy resources with onshore power systems at a very large scale (i.e. making them part of a Super Grid that interconnects distant renewable sources, loads and energy storage systems in a transnational perspective) requires a complete shift in the way the offshore network will be designed and controlled.
Up until now, offshore energy farms have been designed and operated independently from one another, and the corresponding electric grid mostly responds to a ‘point-to-point’ logic. The need for a much higher degree of interconnection in the future will require the interoperability of equipment, which is often provided by different suppliers. Moreover, a different type of optimisation of the entire infrastructure will be required to take into account topology, control and operation synergically.
The first technological shift in offshore transmission systems is already taking place: with the increasing level of produced power and distance from shore, the use of high voltage alternating current (HVAC) to transmit power to shore is becoming untechno-economically viable. Thus, high voltage direct current (HVDC) transmission may be preferred for distances above 100km, as proven by Bard Offshore I, the first offshore wind farm employing HVDC transmission, which was completed in Germany in 2013.
HVDC transmission for offshore applications is, however, an evolving technology that will undergo significant innovation in the close future. This will affect both the single component/equipment (in terms of new topologies, improved performance, increased reliability etc.) and the system level design, especially for the challenges associated with large, meshed configurations.
Newly built offshore grids using HVDC will need to be properly integrated with traditional onshore AC infrastructures, optimising the efficiency of the overall AC/DC system. This will contribute to the evolution of the electrical network from a traditional model, dominated by electromechanical subsystems, to a completely different and ‘smarter’ one. The emerging offshore grid will be characterised by high penetration of power electronics interfaces, high flexibility of operation, and increased sharing of real-time information among all the players involved in the electricity exchange.
Overall, the realisation of an optimised HVDC grid will require at first a wise selection of the topology and layout, going beyond the mere interconnection of existing offshore farms and loads. On the other hand, new control strategies will need to be elaborated in order to guarantee the stable, reliable and efficient operation of the offshore system in all possible practical situations, from normal operation to fault conditions.
In particular, faulty situations will need to be handled in a way that complies with relevant grid codes and at the same time minimises the impact on the electric grid in terms of extension of the power outages. For HVDC grids this will still require significant research on both specific circuit components, such as DC breakers, and suitable strategies for fault handling in multi-terminal and meshed configurations.
The electrification of offshore areas that are presently untouched by grid penetration is simultaneously a challenge and an opportunity. Only the integration of several complementary competencies and the collaboration of different highly skilled professionals will make it possible to hit the target in an optimised way, ensuring long term sustainability.
The Smart Grid and Renewable Energy Laboratory at NTNU
The Department of Electrical Power Engineering at NTNU has been involved in the research field of offshore applications for decades. It has actively investigated both the offshore generation from diversified energy resources (wind, wave, tidal) and the optimisation of offshore transmission and distribution networks, including advanced solutions for power supply to oil and gas platforms.
A key way to confront the challenges of offshore grids is to match theoretical and simulation studies with experimental results obtained from downscaled set-ups in a laboratory environment. Such methodology is essential to test offshore system behavior under a wide variety of controllable and replicable conditions. This ensures critical points can be identified at an early stage of development so that full scale offshore deployment can be carried out only with well-proven technologies and configurations.
The Smart Grid and Renewable Energy Laboratory, jointly operated by NTNU and SINTEF, provides a flexible infrastructure to validate offshore system operation under normal and fault conditions by experimental tests on reduced scale set-ups. Such set-ups can include passive components, electrical machines and power electronics converters in a power range up to 70kVA, with associated control and protection systems. In order to further bridge the gap between simulation studies and experimental validation, the laboratory is also equipped for real-time simulation and power and/or control hardware-in-the-loop testing.
In particular, the Smart Grid and Renewable Energy Laboratory allows for testing the low voltage ride through capability of offshore and onshore energy systems, assessing system performance under transients and unbalanced and/or distorted grid voltage conditions, as well as testing the interoperability of various converter topologies and control systems.
The capabilities of the laboratory are being further extended within the Smart Grid National Laboratory initiative led by NTNU. This will empower the infrastructure by integrating it with the Energy Storage Laboratory, which has the capacity to both test the lifecycle for battery packs and integrate battery energy storage into system-oriented testing of power converter control methods.
Within the same project a high bandwidth grid emulator is being acquired and the capacity for real-time simulation and testing of converters and control systems will be enhanced by remote access to parts of the set-ups from distant locations.
The Smart Grid and Renewable Energy Laboratory is exploited in many national and international projects developed in close collaboration between industry and academia. It is also integrated into the international network of top level infrastructures for the grid integration of marine energy technologies (e.g. Seventh Framework Programme MARINET project), and its technical characteristics and flexibility of use make it a cornerstone of European research in the field of offshore grid development.
Professor Elisabetta Tedeschi
Department of Electric Power Engineering
Norwegian University of Science & Technology
tel: +47 73594240
[email protected]
www.elkraft.ntnu.no